This is part one of a two-part article where we take a look at NAD+ biology, its history, how it relates to the biology of aging, and the potential for therapeutic interventions via the so-called NAD+ precursors.
What is NAD+?
Nicotinamide adenine dinucleotide (NAD) is a coenzyme found in all living cells. It is a dinucleotide, which means that it consists of two nucleotides joined through their phosphate groups. One nucleotide contains an adenine base, and the other contains nicotinamide.
In metabolism, NAD facilitates redox reactions, carrying electrons from one reaction to another. This means that NAD is found in two forms in the cell; NAD+ is an oxidizing agent that takes electrons from other molecules in order to become its reduced form, NADH. NADH can then become a reducing agent that donates the electrons it carries. The transfer of electrons is one of the main functions of NAD, though it also performs other cellular processes, including acting as a substrate for enzymes that add or remove chemical groups from proteins in post-translational modifications.
NAD+ is created from simple building blocks, such as the amino acid tryptophan, and it is created in a more complex way via the intake of food that contains nicotinic acid (niacin) or other NAD+ precursors. These different pathways ultimately feed into a salvage pathway, which recycles them back into the active NAD+ form.
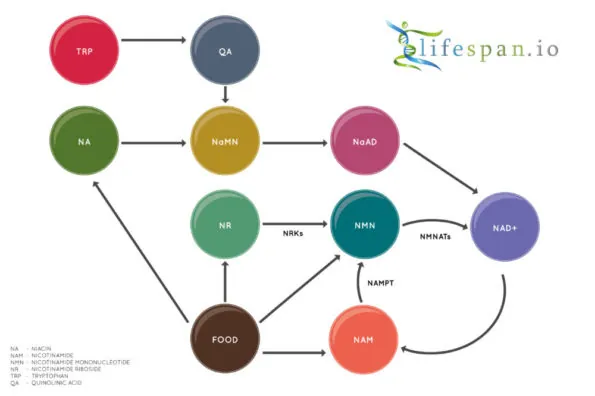
Nicotinamide adenine dinucleotide (NAD+) biology has seen a great deal of interest in the last few years, partially due to the discovery of two precursors of NAD+ biosynthesis, nicotinamide riboside (NR) and nicotinamide mononucleotide (NMN), which both increase NAD+ in multiple tissues.
Accumulating evidence suggests that NAD+ systemically declines with age in a variety of organisms, including rodents and humans, which contributes to the development of many age-related diseases. For this reason, there is a great deal of interest in creating potential interventions that increase NAD+ levels via precursors, thus delaying or even preventing certain aspects of age-related functional decline and diseases.
History of NAD+ research
Before we talk more about NAD+ precursors, let’s take a look at the history of NAD+ biology in order to get a better idea of the background and research that has taken place.
We have known about NAD+ for over a hundred years. Harden and Young originally discovered NAD+ back in 1906, when they discovered that it increased the rate of fermentation in yeast [1]. In the years following this, Euler-Chelpin, a German-born Swedish biochemist, identified that NAD+ was a nucleotide and, in 1929, received the Nobel Prize in Chemistry for his research on the fermentation of sugar and the enzymes involved in the resulting complex reactions.
In 1937, the American biochemist Conrad Elvehjem identified two vitamins, nicotinic acid and nicotinamide, which were deficient in people suffering from pellagra [2]. In 1949, Elvehjem showed that nicotinamide was able to prevent pellagra in dogs by increasing the production of NAD+ [3]. This discovery ended up with nicotinic acid and nicotinamide being classified as vitamin B3, and they are considered to be the original NAD+ precursors.
The first NAD+ gold rush
In 1963, there was a surge of interest in NAD+, when Chambon, Weill, and Mandel showed that NMN activated a previously unknown DNA-dependent polyadenylic acid-synthesizing nuclear enzyme [4]. This research subsequently led to the discovery of poly-ADP-ribose and poly-ADP-ribose polymerases (PARPs), which are part of a family of proteins involved in a number of cellular processes, including DNA repair, maintaining genomic stability, and programmed cell death [5].
In 1965, Hayaishi and his team started to reveal the NAD+ pathway, how it was created via tryptophan, and the NAD+ intermediate nicotinic acid mononucleotide (NMN) [6]. In 1966, Gholson suggested that there was an active turnover cycle of NAD+, which, at the time, performed “an important but as yet unknown function” relating to cellular metabolism [7]. A decade later, in 1976, this was confirmed by Rechsteiner and his team when they published evidence for the rapid turnover of NAD+; this further supported the suggestion that NAD+ had another major function in eukaryotic cells in addition to its established role in oxidation and reduction [8].
In 1989, Lee and his research team incubated NAD+ with extracts from sea urchin eggs, discovering a new NAD+ metabolite, cyclic ADP-ribose [9]. The enzyme ADP-ribosyl cyclase was initially identified in 1991 by Lee and his team in Aplysia, a type of large sea slug [10]. It was later found to exist in mammals as CD38, which was confirmed by Lee, Walseth, and Lee in 1992 [11].
The second NAD+ gold rush
There was another surge of interest in NAD+ biology that happened in the year 2000 and has lasted until the present. The study that started the renewed interest was published by Guarente in 2000, which showed that yeast SIR2 (silent information regulator 2) and the related mouse ortholog, SIRT1, both had NAD+ dependent protein deacetylase activity [12]. Adding further fuel to the fire, in 2001, human nicotinamide/nicotinic acid mononucleotide adenylyltransferase (NMNAT) was successfully isolated [13]. NMNAT is an important NAD+ biosynthetic enzyme, so its isolation and characterization was an important step.
Next, nicotinamide phosphoribosyltransferase (NAMPT), a rate-limiting enzyme responsible for converting nicotinamide into NAD+, was isolated and characterized between 2002-2004 [14-15].
In 2004, nicotinamide riboside was shown to lead to the creation of NAD+ via nicotinamide riboside kinases (NRKs). This study demonstrated that nicotinic acid mononucleotide was not the only path to NAD+ biosynthesis [16].
Is this a third NAD+ gold rush?
While it’s fair to say that interest in NAD+ biology, especially in the context of aging, has not really gone away since the second gold rush period, if anything, the level of interest has skyrocketed in the last few years.
There are now many companies involved in offering supplements based on NAD+ precursors, such as ChromaDex and Elysium Health in the case of nicotinamide riboside along with NOVOS, which offers nicotinamide mononucleotide.
These all aim to increase NAD+ levels, as recent studies appear to suggest that NAD+ systemically declines with age in a variety of organisms, including rodents and humans, which contributes to the development of many age-related diseases. For this reason, there is a great deal of interest in creating potential interventions that increase NAD+ levels via precursors, thus delaying or even preventing certain aspects of age-related functional decline and diseases.
A 2012 study reported that there is a significant age-related decline of NAD+ levels in skin, and while not exact, the average level of concentration seems to fall by a minimum of 50% [17]. The difference between newborns and adults, for example, appears to be several-fold lower, according to the findings of the study.
Regarding the brain, a 2015 study saw researchers measuring intracellular NAD+ and NADH concentrations and the NAD+/NADH ratio in the human brain, detecting the age-dependent changes in NAD contents and the redox state associated with aging [18].
Another study from 2019 also concluded that an age-dependent decline of the NAD+ levels in the brain was also observed [19]. Taken together, these two studies suggest that there is a decline of NAD+ in the brain of between ~10% to ~25% between the period of young adulthood and old age.
Nuchido is another company seeking to address loss of NAD+, but it is taking a slightly different approach to the problem. Rather than trying to increase the amount of precursors available to be converted into NAD+, this company is instead targeting the age-related inflammation that prevents the body from making its own. Nuchido CEO, Dr. Nichola Conlon, recently wrote an article, The Intertwined Nature of NAD+, CD38, and Senescence, which explores the complexity of NAD+ and how it interacts with inflammatory signals.
If you want to find out more about age-related NAD+ decline, we take an in-depth look at the various ways in which this happens in Why NAD+ Declines During Aging.
2020 was a good year for NAD+ research, with a study showing that Niacin increases NAD+ significantly in human trials, at least in aged human muscle tissue [20]. Niacin has been a popular and cheap supplement for many years, and studies like this suggest that this old dog may have a few new tricks.
The quest to restore NAD+ has picked up even more pace in 2021, with a study showing that increasing NAD+ reduces amyloids and benefits mitochondria and that human cells, aged mice, and nematodes all showed similar results [21]. This gives hope for the treatment of age-related amyloidosis and mitochondrial dysfunction, and, as both are a feature of age-related muscle decline, NAD+ restoration may be a potential solution to age related frailty.
That brings us to the end of our potted history of NAD+; almost half a century after the first surge of interest, we are now enjoying a new era of research and discovery in NAD+ biology. Join us for part two of this article, where we delve deeper into the NAD world.
Literature
[1] Harden, A., & Young, W. J. (1906, October). The alcoholic ferment of yeast-juice. Part II.—The coferment of yeast-juice. In Proc. R. Soc. Lond. B (Vol. 78, No. 526, pp. 369-375). The Royal Society.
[2] Simoni, R. D., Hill, R. L., & Vaughan, M. (2002). Copper as an Essential Nutrient and Nicotinic Acid as the Anti-black Tongue (Pelagra) Factor: the Work of Conrad Arnold Elvehjem. Journal of Biological Chemistry, 277(34), e22-e22.
[3] Elvehjem, C. A. (1949). Pellagra: A Deficiency Disease. Proceedings of the American Philosophical Society, 93(4), 335-339.
[4] Chambon, P., Weill, J. D., & Mandel, P. (1963). Nicotinamide mononucleotide activation of a new DNA-dependent polyadenylic acid synthesizing nuclear enzyme. Biochemical and biophysical research communications, 11(1), 39-43.
[5] Herceg, Z., & Wang, Z. Q. (2001). Functions of poly (ADP-ribose) polymerase (PARP) in DNA repair, genomic integrity and cell death. Mutation Research/Fundamental and Molecular Mechanisms of Mutagenesis, 477(1), 97-110.
[6] Ikeda, M., Tsuji, H., Nakamura, S., Ichiyama, A., Nishizuka, Y., & Hayaishi, O. (1965). Studies on the biosynthesis of nicotinamide adenine dinucleotide II. a role of picolinic carboxylase in the biosynthesis of nicotinamide adenine dinucleotide from tryptophan in mammals. Journal of Biological Chemistry, 240(3), 1395-1401.
[7] Gholson, R. K. (1966). The pyridine nucleotide cycle. Nature, 212(5065), 933.
[8] Rechsteiner, M., Hillyard, D., & Olivera, B. M. (1976). Magnitude and significance of NAD turnover in human cell line D98/AH2. Nature, 259(5545), 695.
[9] Lee, H. C., Walseth, T. F., Bratt, G. T., Hayes, R. N., & Clapper, D. (1989). Structural determination of a cyclic metabolite of NAD+ with intracellular Ca2+-mobilizing activity. Journal of Biological Chemistry, 264(3), 1608-1615.
[10] Lee, H. J., Hong, Y. S., Jun, W., & Yang, S. J. (2015). Nicotinamide riboside ameliorates hepatic metaflammation by modulating NLRP3 inflammasome in a rodent model of type 2 diabetes. Journal of medicinal food, 18(11), 1207-1213.
[11] Walseth, T. F., & Lee, H. C. (1992). Similarities in amino acid sequences of Aplysia ADP-ribosyl cyclase and human lymphocyte antigen CD38. Trends in biochemical sciences, 17(12), 495.
[12] Imai, S. I., Armstrong, C. M., Kaeberlein, M., & Guarente, L. (2000). Transcriptional silencing and longevity protein Sir2 is an NAD-dependent histone deacetylase. Nature, 403(6771), 795.
[13] Schweiger, M., Hennig, K., Lerner, F., Niere, M., Hirsch-Kauffmann, M., Specht, T., … & Ziegler, M. (2001). Characterization of recombinant human nicotinamide mononucleotide adenylyl transferase (NMNAT), a nuclear enzyme essential for NAD synthesis. FEBS letters, 492(1-2), 95-100.
[14] Revollo, J. R., Grimm, A. A., & Imai, S. I. (2004). The NAD biosynthesis pathway mediated by nicotinamide phosphoribosyltransferase regulates Sir2 activity in mammalian cells. Journal of Biological Chemistry, 279(49), 50754-50763.
[15] Rongvaux, A., Shea, R. J., Mulks, M. H., Gigot, D., Urbain, J., Leo, O., & Andris, F. (2002). Pre‐B‐cell colony‐enhancing factor, whose expression is up‐regulated in activated lymphocytes, is a nicotinamide phosphoribosyltransferase, a cytosolic enzyme involved in NAD biosynthesis. European journal of immunology, 32(11), 3225-3234.
[16] Bieganowski, P., & Brenner, C. (2004). Discoveries of nicotinamide riboside as a nutrient and conserved NRK genes establish a Preiss-Handler independent route to NAD+ in fungi and humans. Cell, 117(4), 495-502.
[17] Massudi, H., Grant, R., Braidy, N., Guest, J., Farnsworth, B., & Guillemin, G. J. (2012). Age-associated changes in oxidative stress and NAD+ metabolism in human tissue. PloS one, 7(7), e42357.
[18] Zhu, X. H., Lu, M., Lee, B. Y., Ugurbil, K., & Chen, W. (2015). In vivo NAD assay reveals the intracellular NAD contents and redox state in healthy human brain and their age dependences. Proceedings of the National Academy of Sciences, 112(9), 2876-2881.
[19] Bagga, P., Hariharan, H., Wilson, N. E., Beer, J. C., Shinohara, R. T., Elliott, M. A., … & Detre, J. A. (2020). Single-Voxel 1H MR spectroscopy of cerebral nicotinamide adenine dinucleotide (NAD+) in humans at 7T using a 32-channel volume coil. Magnetic Resonance in Medicine, 83(3), 806-814.
[20] Pirinen, E., Auranen, M., Khan, N. A., Brilhante, V., Urho, N., Pessia, A., … & Haimilahti, K. (2020). Niacin cures systemic NAD+ deficiency and improves muscle performance in adult-onset mitochondrial myopathy. Cell Metabolism.
[21] Romani, M., Sorrentino, V., Oh, C. M., Li, H., de Lima, T. I., Zhang, H., … & Auwerx, J. (2021). NAD+ boosting reduces age-associated amyloidosis and restores mitochondrial homeostasis in muscle. Cell reports, 34(3), 108660.