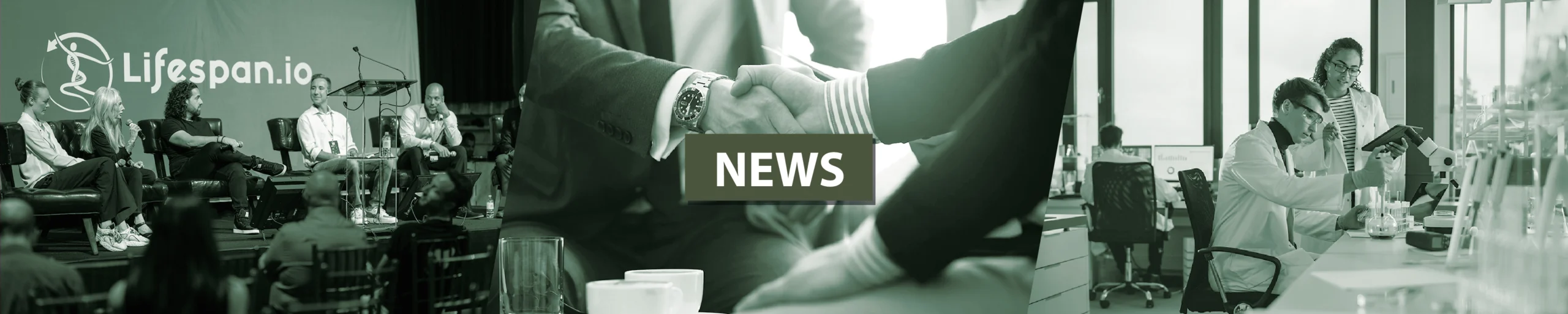
Your #1 Source for Life Extension News
Lifespan.io offers the latest information on rejuvenation biotechnology and life extension technologies. Our news outlet brings you the latest aging research, financial, and advocacy news, which is great if you like to keep up to date with everything happening in this rapidly changing field on a daily basis.
The Latest Longevity News Stories
Fixing Sugar Metabolism Shows Promise Against Dementia
Scientists have shown that aberrant metabolism of glycogen in neurons is linked to the accumulation of harmful tau protein. Caloric restriction, genetic interventions, and small molecules might help [1]. Glycogen and the brain […]
Researchers Connect Cellular Markers to Physical Well-Being
In Aging Cell, a team of researchers has described how the health of skin fibroblasts relates to physical and functional ability. Frailty, capacity, and […]
Healthspan Effects of an Anti-Aging Vaccine on Mice
The researchers of a recent study published in Aging Cell described their novel CD38 peptide vaccine, which improved many measurements of physical health and […]
Study Discovers a Mammalian Mechanism of Tissue Regeneration
Scientists have analyzed the differences between mammalian species that can regrow ear tissue after injury and those that cannot. Their findings can […]
Rejuvenation Roundup June 2025
This month, in between reporting on new methods of affecting aging at its most basic levels, we caught up with AI developers and a longevity fund. Here’s what’s happened in June. LEAF News Springtime for the Longevity Industry: If […]
Researchers Identify a New Dementia Target
Researchers have ascertained that excessive amounts of complement C3, an essential immune protein that increases with aging, are responsible for causing dementia in a mouse model. A necessary complement The complement […]
Subcutaneous and Visceral Fat React Differently to Obesity
Scientists have found that visceral fat and subcutaneous fat produce different responses to obesity in male mice and human patients and identified an important regulator of these processes [1]. The tale of […]
How Part of the Krebs Cycle Affects Senescence
Researchers have discovered how and why α-ketoglutaric acid (AKG) affects cellular senescence and how a small molecule may be useful in affecting this process. They published their findings in Cell Reports. A crucial molecule AKG is a major part of […]
Targeting an Inflammatory Pathway Fights Alzheimer’s
Scientists have discovered that a rare mutation protects against Alzheimer’s disease by dampening a central inflammatory pathway. They recapitulated these results using a small molecule [1]. The woman who beat the odds For […]
Lipid Metabolite Rejuvenates Muscle Stem Cells in Mice
A recent study investigated the effect of a single treatment of prostaglandin E2 on improving muscle strength and rejuvenating muscle stem cells in mice. The researchers explored the molecular and epigenetic aspects underlying this rejuvenation [1]. Aging muscle stem cells […]
Senolytics May Treat Some Long-Term Viral Lung Damage
In Aging Cell, researchers have published their findings that mice exposed to influenza experience long-term consequences that can be partially […]
Longevity Policy, Advocacy in the Spotlight at Vitalist Bay
Just several years ago, longevity conferences were few and far between. Today, there’s no shortage of them. Happening regularly around the globe, they foster scientific debate and […]
Interviewing the leading experts in aging research and longevity
We have a dedicated team of journalists who have interviewed many of the leaders in the field about their research and the drive to end age-related diseases. You can find our latest interviews below.
Regular Digest Articles
We publish the Rejuvenation Roundup – a monthly digest of what is happening in the field, a Longevity Market Recap – a monthly digest focused on the investment and business side of the field, and a quarterly Editorial – focusing on the activities of the news outlet and the wider organization.
Industry Press Releases
You can find some press releases from various companies in our field below. Lifespan.io does not endorse any of these PRs and they are simply provided for information and interest.
Want even more news?
If you want to see even more recent articles, check out all news stories, or if you would like to look at a specific year or month head to the news archive.